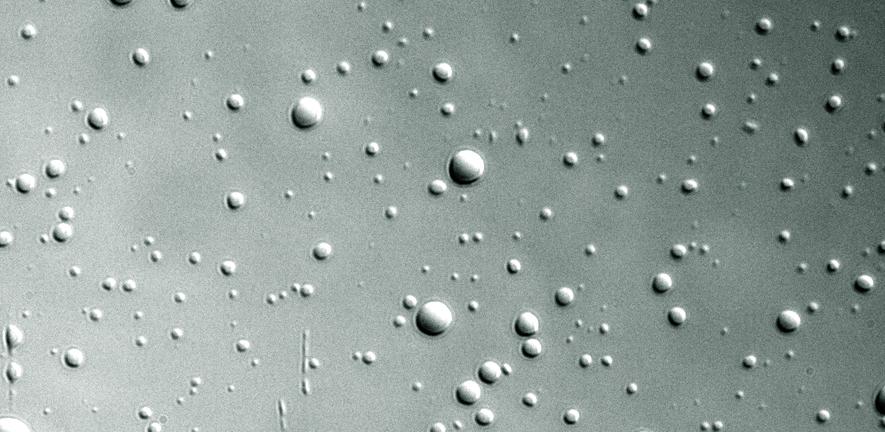
Katherine Stott and colleagues have published a new paper in PNAS that offers insight into the mechanism of DNA condensation and decondensation in eukaryotic cells.
Cryo-electron microscopy (cryo-EM) is transforming the world of structural biology through the imaging of samples at atomic resolution without the need for crystallisation. Despite its widespread use and increasing popularity, some proteins and their complexes will not, however, benefit from the cryo-EM 'resolution revolution'. This is not due to their small size, but rather the fact they are intrinsically disordered and therefore dynamic; dynamic samples often result in structural heterogeneity, which produces smeared or near-invisible density, and the static images obtained of them at cryo-cooled temperatures may not be fully representative of the range of structures sampled at physiological temperatures.
A new class of intrinsically disordered (highly dynamic) proteins has recently come to light, which, surprisingly, retain their disorder and rearrange continually and rapidly even when tightly bound to their interaction partners, making them difficult to study with conventional structural approaches. In their new publication, the Stott team (including prize-winning Part II and III project students from 2017 and 2018, respectively, Oscar Wilkins and Abigail Turner, both now studying for PhDs in London) report the first observation and characterisation of a fully disordered protein in a tight complex with DNA; a discovery that came to light during their experiments with histone H1.
Histone H1 consists of three domains; a central folded 'globular' domain, a short N-terminal region and a long C-terminal tail. It is well-established that the central globular domain seals two turns of DNA around an octamer of core histones to create the nucleosome 'beads-on-a-string' structure, whilst the C-terminal tail is responsible for condensing DNA into fibre-like, higher-order structures. However, the mechanism of DNA condensation is poorly understood, and, despite the key role of histone H1 in this process, scientists are yet to determine the full H1 structure as the C-terminal tail is almost invisible in even the best cryo-EM studies to date due to its highly dynamic nature. Also intriguing is the role of phosphorylation; the C-terminal tail contains phosphorylation sites that are modified throughout the cell cycle, depending on whether the condensed DNA fibre is to be opened up for transcription and replication or rearranged in readiness for mitosis.
The Stott team have now shown, using an armoury of biophysical methods coupled with NMR spectroscopy, that whilst the histone H1-DNA complex is highly disordered, the complexes can self-assemble in vitro into dense liquid droplets (colloidal droplets held together by electrostatic and/or hydrophobic forces, called coacervates) that can be seen under a microscope. The histone H1-DNA complexes within these droplets form higher-order structures in which DNA strands are arranged in a twisted parallel conformation, i.e. a liquid crystal. Phosphorylation of the histone H1 C-terminal tail causes fewer complexes to partition into droplets, but when they do, this higher-order structure is absent.
The authors are cautious here to draw weighty conclusions from their reductionist in vitro approach, but these observations support a similar mechanism to be underpinning DNA fibre formation in vivo, with phosphorylation acting as a tuning process that loosens up the fibre to allow entry of other factors. They speculate that retention of disorder by histone H1 may be a way of reducing unnecessary entropy losses in the DNA-bound state, and also enable H1 to be stably associated to DNA but with its removal readily possible through competing factors that could 'unpeel' the multiple weak interactions cooperating to hold it on to the DNA. Further, the dense liquid droplet state may indeed be an accurate model for the solvent-excluded conditions within the DNA fibre in vivo; the liquidity allowing free diffusion of regulatory molecules in and out.